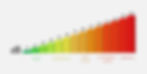
Chances are, you’re fairly familiar with terms such as “decibels” or “decibel units.” If you’ve been working in the arena of architecture or environmental engineering, you’ve probably even incorporated these concepts into your design plan. After all, cultivating a healthy, comfortable, and productive sound environment is as critical to the functionality and appeal of a space as any other design attribute. Using decibel readings to regulate the space’s ambient noise levels is fundamental, then, to the overall design process.
It may come as a surprise, though, to know that the vast majority of people outside of the domain of acoustics really have only an imperfect understanding of what decibels are or how they are measured. Indeed, to the non-expert, it may seem logical that a 20 decibel (dB) sound would be 20 times that of a silent space measuring 0 dBs. In fact, however, a 20 dB sound represents a one hundred-fold amplification of sound.
This is true because decibels are measured not linearly, as with many units of measurement, but logarithmically. That means that even a seemingly small increase in decibel readings can reflect a dramatic increase in noise levels. An accurate and actionable understanding of what decibel measurements truly signify and how they are to be performed is imperative for anyone seeking to cultivate a safe and serene sound environment, whether in the home, workplace, schools, hospitals, government offices, or public spaces.
Linear versus Logarithmic Measurement
As shown above, one of the greatest sources of misunderstanding regarding decibel measurement lies in the assumption that decibel units are linear, as is the case with most other standards of measurement. However, decibel units are calculated logarithmically in order to reflect how the human auditory system actually perceives and processes sound.
The human ear and the auditory processing system are amazingly complex, sophisticated, and dynamic. The human capacity to perceive and process–and tolerate–sound is, fundamentally, logarithmic. Auditory sensitivity decreases, for instance, as sound wave pressures (i.e. volume or amplitude) increase. Thus, hearing is more acute in low-noise environments.
The logarithmic decibel scale reflects the exponential increases in perceived noise levels with every additional decibel. More specifically, sound energy, or perceived volume, doubles for every 3 dB increase in sound. For every 10 dB increase, sound energy increases by a factor of 10, while, as mentioned earlier, every 20 dB increase results in sound energy increasing by a factor of 100!
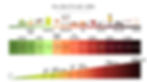
This means that while the normal volume of human conversation in typical sound environments hovers around a comfortable 60 dB, an increase of just 20 decibels can put you into the danger zone, given the immense amplification of sound those few decibels represent.
For this reason, most federal, state, and international institutions generally prohibit daily exposure to noise environments above an average of around 80 dB without the use of protective equipment or other noise mitigation efforts. In the United States, for example, OSHA has set 90 decibels as the maximum permissible exposure level (PEL) per eight-hour workday. Employers in workplaces with an average daily decibel rating of 85 or higher must, according to OSHA regulations, institute a hearing conservation program for all employees.
Perhaps not surprisingly, those expectation-defying decibels can be challenging to measure, particularly when an environment has multiple sound sources (as almost all do). For instance, if you are in a factory with a large industrial machine producing 100 dB of sound and you add another machine generating 100 dB, you’re not going to end up with a noise environment of 200 dB. Rather, you will end up with 103 dB. That’s because you’ve doubled the sound pressure and, according to the logarithmic decibel scale, that means you’ve added 3 dB to the environment.
Why There Are Multiple Decibel Scales
It’s not only in the use of the logarithms that the decibel scale may defy the layperson’s expectations. There are, actually, many decibel scales, not one. Differentiating between them may not be a skill most people will find themselves incorporating into their daily lives, of course. However, for those charged with constructing healthy and safe sound environments, understanding core distinctions between the scales is critical to your efforts. This is because scale differentials enable decibels to be understood as indicators of sound pitch as well as volume. The distinct decibel scales, in other words, measure both the height (amplitude/volume) and the length (frequency/pitch) of soundwaves.
While there are actually four distinct decibel scales, categorized as A-D, scales A and C are the most commonly used, particularly for measuring noise levels in built environments, including workplaces, schools, hospitals, and other public spaces, such as airports. The particular scale used in a decibel measurement is indicated by the relevant letter. Thus, a dBA measurement indicates decibels on the A scale, which attempts to mimic the curve of how human ears hear sound, and pertains to mid-range sound frequencies that fall within the ordinary range of human auditory perception (typically between 500 Hz and 6 kHz).
The dBC scale, conversely, measures lower and higher frequency sounds. Thus, the C scale is typically used to measure sound pressures at lower pitches, such as the “hum” of power lines and other low-frequency ambient noises.
The C scale is significant in spaces such as amphitheaters, where musical instruments produce deep bass notes. Additionally, the measurement can be used to explore a still relatively little understood aspect of acoustic science and human physiology: the impacts of low-frequency sound on human health and wellness. There is mounting evidence that chronic exposure to low-frequency sounds can be as detrimental as exposure to high-volume sounds. Studies suggest that exposure to low-frequency noise, such as that produced by wind turbines, high-voltage power lines, and HVAC systems, can have detrimental impacts on cognition, memory, information processing, emotional and psychological well-being, and cardiac and metabolic functioning (1-5).
Measuring Decibels with the Appropriate Dosimeter For Your Needs
To effectively measure sound in an environment requires good instruments and skill. Depending on your purpose in assessing sound levels, it is possible to acquire noise meters that are reasonably accurate, affordable, and user-friendly. Indeed, a number of noise measuring (or dosimeter) apps are available for iOS or Android and are reliable enough for general use. Some, such as the NIOSH, even offer professional-quality features and are recommended for some commercial uses by institutions such as the CDC and OSHA.
While there is a wide array of sound measurement technology on the market today, not all are created equal. For higher-level needs, caution must be exercised. Some smartphone technologies, particularly those designed for iOS systems, can meet most national and occupational minimum standards for accuracy in noise exposure readings, which is generally +/- 2dBA. Many android-based dosimeter apps are incapable of reaching even these baseline standards. However, if you need a professional quality dosimeter, smartphone apps will not meet your needs.
Instead, when you have a professional project that requires high-level acoustics monitoring, then a standalone sound meter is far and away your best option. Professional quality sound meters are typically assorted into two classes.
Class 2 meters are designed to meet most industry standards but are generally less accurate than their Class 1 counterparts. Class 1 meters are the gold standard in accuracy and reliability and are designed to meet rigorous industry standards.
However, to optimize accuracy, both Class 1 and Class 2 meters must be routinely recalibrated in an accredited acoustics laboratory. In addition, meter functionality must be evaluated after each day of use with a precision calibration instrument to ensure their accuracy.
As might be expected, the costs of these devices will vary widely. General use dosimeter apps for smartphones, which are not calibrated, can cost as little as a few dollars. Likewise, uncalibrated, general use, handheld meters average around $100, but they typically do not meet industry accuracy standards. Professional quality sound meters, on the other hand, can cost hundreds of dollars for a Class 2 instrument and up to several thousands of dollars for a Class 1 meter.
Determining what kind of dosimeter you need will depend very much on your purposes. If, for instance, you are an acoustical engineer or an acoustic designer and you are engaged in high-level sound mitigation efforts (i.e. for healthcare, academic, or industrial settings), then a Class 1 meter is likely to be worth the investment. These can provide the peace of mind of knowing you are meeting industry and national standards, fulfilling contractual obligations, and adhering to client specifications.
Regardless of which instrument(s) you choose, however, it’s important to bear in mind that even the most sophisticated meter is only as accurate as the calibration and testing it is subjected to. And that requires routine access to an accredited acoustics lab.
Why Sound Measurement Matters
To be sure, maintaining a comfortable sound environment is central to an individual’s experience of the space. But mitigating environmental noise is about far more than ensuring the space is pleasing to the senses.
Regulating noise levels, above all, is critical to physical and mental health. Studies have shown that prolonged exposure to environmental noise significantly increases the risk of a host of physiological and psychological illnesses, ranging from depression and anxiety to metabolic and cardiovascular disease and even dementia (6-10).
Indeed, there is mounting evidence that the impacts of environmental noise exposure on human health and functioning are significant and long-lasting. Because of this, architects, environmental engineers, and urban planners are placing increasing emphasis on noise mitigation in schools, workplaces, and healthcare facilities.
How FSorb Can Help
At FSorb, we understand the critical importance of a sound environment on human health, happiness, and functioning. That’s why we offer a wide array of innovative and highly customizable sound mitigation products to suit every environment, from schools and hospitals to factories, offices, and apartment complexes. Contact your local FSorb representative today to discuss how our sound treatment solutions can help you in your next sound design project.
At FSorb, we are motivated by improving human health and do so by creating eco-friendly acoustic products. Our mission is to help designers build beautiful spaces that reduce excess ambient noise while calming the human nervous system. With over 25 years in the acoustic business we stand behind FSorb as a durable, environmentally friendly, and low-cost product. If you want an acoustic solution that is safe to human health at an affordable price, then we are your resource.
info@fsorb.com
(844) 313-7672
Sources:
Chiu CH, Lung SC, Chen N, Hwang JS, Tsou MM. Effects of low-frequency noise from wind turbines on heart rate variability in healthy individuals. Sci Rep. 2021 Sep 8;11(1):17817. doi: 10.1038/s41598-021-97107-8. PMID: 34497296; PMCID: PMC8426498.
Carlile S, Davy JL, Hillman D, Burgemeister K. A Review of the Possible Perceptual and Physiological Effects of Wind Turbine Noise. Trends Hear. 2018 Jan-Dec;22:2331216518789551. doi: 10.1177/2331216518789551. PMID: 30084316; PMCID: PMC6081752.
Javadi A, Pourabdian S, Forouharmajd F. The Effect of Low Frequency Noises Exposure on the Precision of Human at the Mathematical Tasks. Int J Prev Med. 2022 Feb 23;13:33. doi: 10.4103/ijpvm.IJPVM_244_20. PMID: 35392306; PMCID: PMC8980828.
Alves JA, Silva LT, Remoaldo PC. Impacts of low frequency noise exposure on well-being: a case-study from portugal. Noise Health. 2018 Jul-Aug;20(95):131-145. doi: 10.4103/nah.NAH_64_17. PMID: 30136673; PMCID: PMC6122264.
Takahashi Y. [Present situation and research task on the assessment of psychological effects caused by low-frequency noise]. Nihon Eiseigaku Zasshi. 2013;68(2):88-91. Japanese. doi: 10.1265/jjh.68.88. PMID: 23718970.
Sivakumaran K, Ritonja JA, Waseem H, AlShenaibar L, Morgan E, Ahmadi SA, Denning A, Michaud DS, Morgan RL. Impact of Noise Exposure on Risk of Developing Stress-Related Health Effects Related to the Cardiovascular System: A Systematic Review and Meta-Analysis. Noise Health. 2022 Jul-Sep;24(114):107-129. doi: 10.4103/nah.nah_83_21. PMID: 36124520; PMCID: PMC9743313.
Yadav OP, Sarkar A, Shan D, Rahman A, Moro L. Occupational noise exposure and health impacts among fish harvesters: a systematic review. Int Marit Health. 2021;72(3):199-205. doi: 10.5603/IMH.2021.0038. PMID: 34604990.
Apparicio P, Gelb J, Jarry V, Lesage-Mann É. Cycling in one of the most polluted cities in the world: Exposure to noise and air pollution and potential adverse health impacts in Delhi. Int J Health Geogr. 2021 Apr 30;20(1):18. doi: 10.1186/s12942-021-00272-2. PMID: 33931110; PMCID: PMC8086121.
Wang SS, Glied S, Williams S, Will B, Muennig PA. Impact of aeroplane noise on mental and physical health: a quasi-experimental analysis. BMJ Open. 2022 May 2;12(5):e057209. doi: 10.1136/bmjopen-2021-057209. PMID: 35501087; PMCID: PMC9062823.
Hegewald J, Schubert M, Freiberg A, Romero Starke K, Augustin F, Riedel-Heller SG, Zeeb H, Seidler A. Traffic Noise and Mental Health: A Systematic Review and Meta-Analysis. Int J Environ Res Public Health. 2020 Aug 25;17(17):6175. doi: 10.3390/ijerph17176175. PMID: 32854453; PMCID: PMC7503511.