Acoustics in Architecture: Fundamental Principles Architects, Designers, & Contractors Need to Know
- FSorb
- Oct 11, 2022
- 9 min read
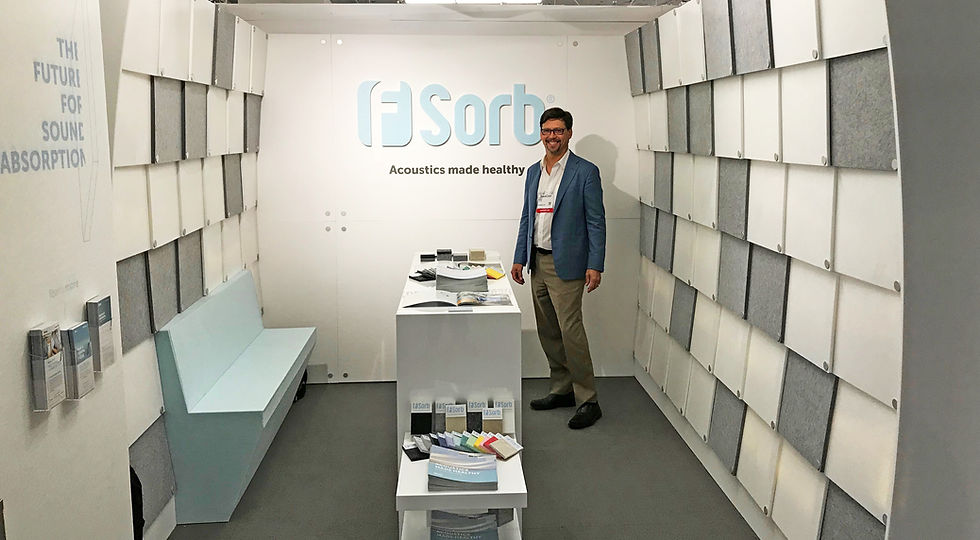
Whether you’re designing a residential property, a commercial space, or a public building, your goal may be to create an environment that is both highly functional and deeply pleasing to the senses. Achieving this goal is an art and science, calling on you to join the technical with the aesthetic.
That means that you must draw on a myriad of tools from your designer’s toolbox. However, there’s one element of design with which you may be less familiar, one element that is often overlooked in your professional practice. It’s acoustics, and it’s a science and a strategy that can make or break how people experience your design.
This article discusses some of the most important concepts in acoustic design, and reminders for architects, interior designers, and contractors to design spaces that are comfortable and easy on the nervous system.
A Quick Disclaimer
This guide is not intended to replace a good acoustical engineer. The science of acoustics is vast and profoundly complex, deeply rooted in the fields of physics and applied mathematics. This guide has been written to provide insights and reminders for design considerations. Our hope is that these fundamental concepts will help you design residential, commercial, and public acoustically pleasing spaces that sound as good as they look.
The Significance of the Sound Environment
Given all that designers must address when creating spaces that are both functional and comfortable, you may be wondering why sound should take precedence. Isn’t this a relatively easy fix and, or an issue that the building’s future occupants can worry about?
Well, not exactly, because many aspects of the sound quality of a space are already built into the environment, before those who will use the space ever lay an eye–or an ear–on it. The design and the construction of the environment will determine how noisy or quiet it is in ordinary conditions, and that will determine its overall functionality. For example, hard surfaces bounce sound around, and this sound needs to be prevented from entering other spaces while also being absorbed within each space. Attention to design can greatly impact both aspects, allowing you to create acoustically pleasing spaces that do not stress the nervous system with excess noise.
Improving the sound environment is important because it impacts the nervous system and plays an instrumental role in the health, happiness, and overall functioning of those in it. Indeed, exposure to environmental noise (i.e. to sounds that are considered to be extraneous, disruptive, or unwanted by the hearer) can take a profound toll on academic and workplace performance and on physical and mental health.
For example, research shows that patients exposed to noisy hospital environments are at risk for poorer outcomes, including an increased likelihood of stress-related complications and mortality (2, 3, 4, 5, 6).
There is also quite a bit of evidence proving that prolonged exposure to environmental noise, such as that produced by air and ground traffic, crowded urban environments, or industrial machinery, can increase the risk of chronic and often life-threatening illnesses, from cardiovascular disease to dementia to major depressive disorder (7, 8, 9, 10, 11).
Noise pollution can also rob those exposed to it of the capacity to achieve their full potential. Poor sound environments in schools and workplaces have been associated with a range of detrimental impacts on cognitive performance, including challenges with memory, concentration, attention, and information processing and retention (12, 13, 14, 16, 17, 18).
The implications for architects, engineers, designers, and contractors of this increasing body of knowledge surrounding the role of the sound environment in the experience and functionality of the built environment are clear. Whether you are building a school, a healthcare facility, an office building, or a private home, if you want the space to be safe, comfortable, pleasing, and functional for occupants, then it is important to prioritize acoustic design as an integral component of design.
The Science of Sound
In order to understand the basics of acoustic design, you need to know first how sound works, how it moves, and why it matters. In essence, sound is energy generated by a source and traveling in waves until it reaches a medium that absorbs or deflects it.
In internal environments, of course, there can be many sources of sound, and those
sounds are often emitted simultaneously. Thus, you may have human speakers competing with footsteps, animals making noises, machines humming and buzzing, and with walls, ceilings, floors, and other surfaces reverberating right along with them.
Sound frequency
In other words, there can be a wide array of sound energy impulses circulating through an enclosed space at the same time. The medium through which they travel is the air, and the receivers are the various living ears, both humans and non-human animals. But those are not the only receivers of sound energy: apertures, such as doors, windows, and cracks and crevices within a room, receive and, often, transmit sound energy, while surfaces, such as walls, floors, ceilings, and objects in the room receive and then absorb, reflect, or transmit it.
Sound energy moves through a medium (i.e. air or water) in the form of waves. The wavelength, which refers to the distance between the peaks of a wave, will determine the frequency of a sound. Another way to understand frequency is to consider the speed at which a sound wave oscillates. Longer wavelengths will strike a receiver at a lower speed, meaning there are significantly fewer oscillations or vibrations striking the receiver per second than sounds with shorter wavelengths and faster oscillations.
Low pitch (low frequency) sounds have a longer wavelength than high pitch (high frequency) sounds. Low frequencies, in general, are also more difficult for humans to detect than mid and high frequencies. Typically, a low frequency sound is considered to fall below the 200 hertz (Hz) range, while anything above 2000 Hz is generally classified as high frequency.
Though most humans with normal hearing are most sensitive to mid and high frequency sounds, the human ear can perceive an extraordinary range of frequencies. While frequency perception tends to decline with age, most young adults with normal hearing can detect sounds as low as 60 Hz and as high as 20,000 Hz.
Wave Amplitude
Sound waves don’t just determine the pitch of a sound, they also determine the sound’s volume. While the distance between the peaks of a wave or the speed of wave oscillations determines sound frequency, the height of the peaks of the sound wave determines the sound’s loudness. This is referred to as amplitude, and it is measured in decibels (dB).
In normal conditions, a human voice speaking at an ordinary conversational volume will average less than 60 dBs. Environmental sounds that may be considered loud and disruptive but not harmful for a short exposure (such as the sound of a vacuum cleaner) typically produce around 70 dBs of sound.
However, sounds that surpass the 85 dBs threshold, such as that produced by most industrial equipment, large vehicles, or aircraft, can inflict significant damage with long-term exposure. Federal statutes regarding workplace noise exposure, such as those defined by OSHA, typically require harm reduction practices, including employee training, the provision of sound protection equipment, and environmental sound mitigation, when average environmental noise levels exceed 85 dBs.
Built Environments and the Movement of Soundwaves
Not surprisingly, it isn’t just the frequency and amplitude of sound waves that determine the sound environment of a space. The way sound waves move through a medium (i.e. air) and what happens when the wave strikes a surface also affect the soundscape.
Soundwave motion and the (literal) impact of the wave on the receiver are, in turn, influenced by a host of factors, such as the relative position of the sound source to the receiver; the temperature, humidity levels, and barometric pressure of the air through which the soundwaves travel; the quantity, shape, and placement of objects in the environment, and the shape of the room, its relationship to adjacent rooms, and the materials from which surfaces in the room are made.
It is these latter considerations, the placement, shape, type, and material composition of every surface in the environment, that have the greatest impact on the space’s sound environment. This is because, when a sound wave strikes a surface, only one of three things can happen: either the wave is absorbed, or it is transmitted through the surface, or it is reflected off the surface and back into the environment.
Far more often than not, some combination of all three occurs, depending on the shape of the surface, the position of the surface relative to the source of the sound, and the type of materials from which the surface is made.
For instance, straight, flat surfaces made of dense materials and positioned perpendicularly to the sound source reflect more energy from sound waves than they absorb. This is particularly true in large open spaces, such as gymnasiums.
On the other hand, porous materials are generally better at absorbing sound waves,
particularly on flat surfaces. Curved walls, conversely, do little to mitigate sound wave reflection because the angle contributes to a concentration of reflected soundwaves in more localized areas, producing echoes. This is why auditoriums featuring domed ceilings or other concave surfaces generally require substantial sound mitigation treatment to optimize environmental sound quality by breaking up and dispersing sound waves before they can be reflected back into the environment (1).
Some designers incorporate plants for both aesthetics and to absorb sound. It is interesting to note, though, that it is a myth that nature will block noises effectively, especially inside a building. Leaves from a tree and vegetation do not block sound and provide minimal absorption.
While there are elements of environmental sound quality that are beyond the control of the architect, designer, and contractor, there are also a host of factors that are within their scope. This includes, at a minimum, the construction and design of the space and the selection of materials. Much more on this in the second part of this two-part series!
At FSorb, we are motivated by improving human health and do so by creating eco-friendly acoustic products. Our mission is to help designers build beautiful spaces that reduce excess ambient noise while calming the human nervous system. With over 25 years in the acoustic business we stand behind FSorb as a durable, environmentally friendly, and low-cost product. If you want an acoustic solution that is safe to human health at an affordable price, then we are your resource.
info@fsorb.com
(844) 313-7672
Sources:
Vercammen, M. L. (2013). Sound concentration caused by curved surfaces. Proceedings of meetings on acoustics. 19. https://asa.scitation.org/doi/abs/10.1121/1.4800250
de Lima Andrade E, da Cunha E Silva DC, de Lima EA, de Oliveira RA, Zannin PHT, Martins ACG. Environmental noise in hospitals: a systematic review. Environ Sci Pollut Res Int. 2021 Apr;28(16):19629-19642. doi: 10.1007/s11356-021-13211-2. Epub 2021 Mar 5. PMID: 33674976; PMCID: PMC7935697.
Joseph BE, Mehazabeen H, U M. Noise pollution in hospitals - A study of public perception. Noise Health. 2020 Jan-Mar;22(104):28-33. doi: 10.4103/nah.NAH_13_20. PMID: 33243965; PMCID: PMC7986457.
Rodarte MDO, Fujinaga CI, Leite AM, Salla CM, Silva CGD, Scochi CGS. Exposure and reactivity of the preterm infant to noise in the incubator. Codas. 2019 Nov 7;31(5):e20170233. Portuguese, English. doi: 10.1590/2317-1782/20192017233. PMID: 31721912.
Singh D, Fusch G. Investigating Noise Exposure to Newborn Infants From Respiratory Support: Methodological Considerations. Cureus. 2021 Nov 8;13(11):e19353. doi: 10.7759/cureus.19353. PMID: 34909314; PMCID: PMC8653757.
Hutchinson G, Du L, Ahmad K. Incubator-based Sound Attenuation: Active Noise Control In A Simulated Clinical Environment. PLoS One. 2020 Jul 15;15(7):e0235287. doi: 10.1371/journal.pone.0235287. PMID: 32667931; PMCID: PMC7363066.
Hahad O, Bayo Jimenez MT, Kuntic M, Frenis K, Steven S, Daiber A, Münzel T. Cerebral consequences of environmental noise exposure. Environ Int. 2022 Jul;165:107306. doi: 10.1016/j.envint.2022.107306. Epub 2022 May 20. PMID: 35635962.
Münzel T, Schmidt FP, Steven S, Herzog J, Daiber A, Sørensen M. Environmental Noise and the Cardiovascular System. J Am Coll Cardiol. 2018 Feb 13;71(6):688-697. doi: 10.1016/j.jacc.2017.12.015. PMID: 29420965.
Cantuaria ML, Waldorff FB, Wermuth L, Pedersen ER, Poulsen AH, Thacher JD, Raaschou-Nielsen O, Ketzel M, Khan J, Valencia VH, Schmidt JH, Sørensen M. Residential exposure to transportation noise in Denmark and incidence of dementia: national cohort study. BMJ. 2021 Sep 8;374:n1954. doi: 10.1136/bmj.n1954. PMID: 34497091; PMCID: PMC8424489.
Yu Y, Mayeda ER, Paul KC, Lee E, Jerrett M, Su J, Wu J, Shih IF, Haan M, Ritz B. Traffic-related Noise Exposure and Late-life Dementia and Cognitive Impairment in Mexican-Americans. Epidemiology. 2020 Nov 1;31(6):771-778. doi: 10.1097/EDE.0000000000001249. PMID: 33555809; PMCID: PMC8627325.
Dzhambov AM, Lercher P. Road Traffic Noise Exposure and Depression/Anxiety: An Updated Systematic Review and Meta-Analysis. Int J Environ Res Public Health. 2019 Oct 27;16(21):4134. doi: 10.3390/ijerph16214134. PMID: 31717834; PMCID: PMC6862094.
Alimohammadi I, Kanrash FA, Abolghasemi J, Vosoughi S, Rahmani K, Chalak MH. Relationship Between Noise Annoyance and Cognitive Performance in Automotive Workers Exposed to Chronic Noise. J UOEH. 2019;41(4):375-385. doi: 10.7888/juoeh.41.375. PMID: 31866655.
Irgens-Hansen K, Gundersen H, Sunde E, Baste V, Harris A, Bråtveit M, Moen BE. Noise exposure and cognitive performance: A study on personnel on board Royal Norwegian Navy vessels. Noise Health. 2015 Sep-Oct;17(78):320-7. doi: 10.4103/1463-1741.165057. PMID: 26356374; PMCID: PMC4900491.
Shield BM, Dockrell JE. The effects of environmental and classroom noise on the academic attainments of primary school children. J Acoust Soc Am. 2008 Jan;123(1):133-44. doi: 10.1121/1.2812596. PMID: 18177145.
Chere B, Kirkham N. The Negative Impact of Noise on Adolescents' Executive Function: An Online Study in the Context of Home-Learning During a Pandemic. Front Psychol. 2021 Sep 22;12:715301. doi: 10.3389/fpsyg.2021.715301. PMID: 34630225; PMCID: PMC8492971.
Pfeiffer B, Stein Duker L, Murphy A, Shui C. Effectiveness of Noise-Attenuating Headphones on Physiological Responses for Children With Autism Spectrum Disorders. Front Integr Neurosci. 2019 Nov 12;13:65. doi: 10.3389/fnint.2019.00065. PMID: 31798424; PMCID: PMC6863142.
Adamson LB, Bakeman R, Suma K, Robins DL. Autism Adversely Affects Auditory Joint Engagement During Parent-toddler Interactions. Autism Res. 2021 Feb;14(2):301-314. doi: 10.1002/aur.2355. Epub 2020 Aug 18. PMID: 32809260; PMCID: PMC7878296.
Hsieh JJ, Nagai Y, Kumagaya SI, Ayaya S, Asada M. Atypical Auditory Perception Caused by Environmental Stimuli in Autism Spectrum Disorder: A Systematic Approach to the Evaluation of Self-Reports. Front Psychiatry. 2022 Jun 9;13:888627. doi: 10.3389/fpsyt.2022.888627. PMID: 35770058; PMCID: PMC9236639.